Difference between revisions of "Endurance Adaptations"
User:Fellrnr (User talk:Fellrnr | contribs) |
User:Fellrnr (User talk:Fellrnr | contribs) |
||
(3 intermediate revisions by the same user not shown) | |||
Line 2: | Line 2: | ||
There are various adaptations to endurance training, which are discussed in some detail below. Understanding these adaptations can be useful background when looking at the different types of training. (Except where noted, this list is based on "Physiology of sport and exercise"<ref>Jack H. Wilmore, David L. Costill, W. Larry. Kenney, Physiology of sport and exercis, 2008 !!date!!, Human Kinetics !!publisher!!, Champaign, IL !!location!!, isbn 0-7360-5583-5</ref>.) | There are various adaptations to endurance training, which are discussed in some detail below. Understanding these adaptations can be useful background when looking at the different types of training. (Except where noted, this list is based on "Physiology of sport and exercise"<ref>Jack H. Wilmore, David L. Costill, W. Larry. Kenney, Physiology of sport and exercis, 2008 !!date!!, Human Kinetics !!publisher!!, Champaign, IL !!location!!, isbn 0-7360-5583-5</ref>.) | ||
=Heart Adaptations= | =Heart Adaptations= | ||
− | * The heart becomes larger in response to endurance training, with the left side that sends blood to the body having a thicker wall and a larger interior volume. | + | * The heart becomes larger in response to endurance training, with the left side that sends blood to the body having a thicker wall and a larger interior volume. |
* The stroke volume (blood pumped with each beat) also increases. The volume is greater at rest and during exercise, with the stroke volume in a highly trained athlete twice that of an untrained person. This increase is due to multiple factors: | * The stroke volume (blood pumped with each beat) also increases. The volume is greater at rest and during exercise, with the stroke volume in a highly trained athlete twice that of an untrained person. This increase is due to multiple factors: | ||
** The increased heart size. | ** The increased heart size. | ||
− | ** A slower | + | ** A slower [[Heart Rate]] for a given exercise level which allows more time for the heart to fill. |
** Increased total blood volume. | ** Increased total blood volume. | ||
** A greater force of contraction. | ** A greater force of contraction. | ||
− | ** A lower volume of blood remaining in the heart after contraction. | + | ** A lower volume of blood remaining in the heart after contraction. |
− | * [[Resting Heart Rate| Resting Heart Rate]] drops with training. A sedentary person with a resting | + | * [[Resting Heart Rate| Resting Heart Rate]] drops with training. A sedentary person with a resting [[Heart Rate]] of 80 BPM may be able to reduce their resting [[Heart Rate]] by 1 BPM per week of training for up to 10 weeks, though some studies have shown much smaller changes (5 BPM over 20 weeks). |
− | * The [[Heart Rate| Heart Rate]] for a given exercise intensity is reduced, and sedentary people undergoing 6 months of training can reduce the | + | * The [[Heart Rate| Heart Rate]] for a given exercise intensity is reduced, and sedentary people undergoing 6 months of training can reduce the [[Heart Rate]] for a given intensity by 10-30 BPM. |
− | * [[Maximum Heart Rate| Maximum Heart Rate]] does not typically change with endurance training, but several studies have suggested that sedentary people with a Max HR of 180+ BPM may have a slightly reduced value post-training. This reduction in Max HR may be to preserve the time for the heart to refill with blood. Also, older athletes may have less of an age related decline in Max HR than those that are sedentary. | + | * [[Maximum Heart Rate| Maximum Heart Rate]] does not typically change with endurance training, but several studies have suggested that sedentary people with a Max HR of 180+ BPM may have a slightly reduced value post-training. This reduction in Max HR may be to preserve the time for the heart to refill with blood. Also, older athletes may have less of an age related decline in Max HR than those that are sedentary. |
− | * Cardiac output at rest and at moderate intensity exercise remains largely unchanged with training, which is the result of a greater stroke volume at a lower | + | * Cardiac output at rest and at moderate intensity exercise remains largely unchanged with training, which is the result of a greater stroke volume at a lower [[Heart Rate]]. (Sometimes the cardiac output will be slightly lower, probably due to greater oxygen extraction by the muscles.) However, at higher intensity exercise the cardiac output is increased, which results in improved performance. |
=Circulation Adaptations= | =Circulation Adaptations= | ||
− | * Endurance training results in an increased number of capillaries in the muscles. The ratio of capillaries to muscle fibers allows for a greater delivery of blood to the working muscles. In addition, the use of existing capillaries is improved with training. | + | * Endurance training results in an increased number of capillaries in the muscles. The ratio of capillaries to [[Muscle|muscle fibers]] allows for a greater delivery of blood to the working muscles. In addition, the use of existing capillaries is improved with training. |
− | * The human body does not have enough blood to fully supply all capillaries in the body, and so it will redistribute the blood flow to the most needed areas. Endurance training improves this redistribution, including redistribution within the muscles themselves, providing more blood to the slow twitch fibers and less to the fast twitch fibers. | + | * The human body does not have enough blood to fully supply all capillaries in the body, and so it will redistribute the blood flow to the most needed areas. Endurance training improves this redistribution, including redistribution within the muscles themselves, providing more blood to the slow twitch fibers and less to the fast twitch fibers. |
* Blood pressure at a given exercise intensity is reduced, but at maximum intensity systolic pressure is increased and diastolic is reduced. (Systolic is the higher pressure when the heart beats and diastolic is the lower pressure when the heart is refilling.) | * Blood pressure at a given exercise intensity is reduced, but at maximum intensity systolic pressure is increased and diastolic is reduced. (Systolic is the higher pressure when the heart beats and diastolic is the lower pressure when the heart is refilling.) | ||
* Endurance training generally lowers resting blood pressure. Systolic is reduced by ~4 mm Hg and diastolic by 3 mm Hg in people with either high or normal blood pressure<ref name="Whelton-2002">SP. Whelton, A. Chin, X. Xin, J. He, Effect of aerobic exercise on blood pressure: a meta-analysis of randomized, controlled trials., Ann Intern Med, volume 136, issue 7, pages 493-503, Apr 2002, PMID [http://www.ncbi.nlm.nih.gov/pubmed/11926784 11926784]</ref>. | * Endurance training generally lowers resting blood pressure. Systolic is reduced by ~4 mm Hg and diastolic by 3 mm Hg in people with either high or normal blood pressure<ref name="Whelton-2002">SP. Whelton, A. Chin, X. Xin, J. He, Effect of aerobic exercise on blood pressure: a meta-analysis of randomized, controlled trials., Ann Intern Med, volume 136, issue 7, pages 493-503, Apr 2002, PMID [http://www.ncbi.nlm.nih.gov/pubmed/11926784 11926784]</ref>. | ||
− | * Blood volume increases with endurance training, and higher intensity produces a greater change. | + | * Blood volume increases with endurance training, and higher intensity produces a greater change. |
− | ** Blood plasma increases rapidly, with initial changes seen within an hour of the end of the first bout of training. | + | ** Blood plasma increases rapidly, with initial changes seen within an hour of the end of the first bout of training. |
− | ** The number of red blood cells, that carry oxygen in the blood, also increases with training. While these extra cells add to the increase in overall blood volume, because the plasma increases disproportionately more than the red blood cells, the net effect is that the blood becomes more dilute and can result in a false indication of anemia (pseudoanemia). Having thinner blood may be an advantage to an athlete, as it will flow more easily through the capillaries. | + | ** The number of red blood cells, that carry oxygen in the blood, also increases with training. While these extra cells add to the increase in overall blood volume, because the plasma increases disproportionately more than the red blood cells, the net effect is that the blood becomes more dilute and can result in a false indication of anemia (pseudoanemia). Having thinner blood may be an advantage to an athlete, as it will flow more easily through the capillaries. |
* The oxygen saturation of blood returning from the exercising body to the heart is lowered after training. This is due to an improved ability of the muscles to utilize the supplied oxygen | * The oxygen saturation of blood returning from the exercising body to the heart is lowered after training. This is due to an improved ability of the muscles to utilize the supplied oxygen | ||
=Lung (pulmonary) Adaptations= | =Lung (pulmonary) Adaptations= | ||
− | * Generally, there is little structural adaptation of the lungs, as their capacity is far greater than is needed in most individuals. | + | * Generally, there is little structural adaptation of the lungs, as their capacity is far greater than is needed in most individuals. |
− | * Breathing at rest is unchanged with training, but for a given sub-maximal intensity, | + | * [[Breathing]] at rest is unchanged with training, but for a given sub-maximal intensity, [[Breathing]] is reduced by as much as 20-30%. Maximal [[Breathing]] increases with training, with increases in both the [[Breathing]] rate and volume. |
− | * In some highly trained athletes the lungs are unable to keep up at high exercise intensities and the blood oxygen saturation ([[SpO2|SpO<sub>2</sub>]]) can drop. | + | * In some highly trained athletes the lungs are unable to keep up at high exercise intensities and the blood oxygen saturation ([[SpO2|SpO<sub>2</sub>]]) can drop. |
− | * Training increases the blood flow to the lungs (perfusion), which combined with greater | + | * Training increases the blood flow to the lungs (perfusion), which combined with greater [[Breathing]] and lower oxygen saturation of the blood entering the lungs results in a greater uptake of oxygen (pulmonary diffusion). |
=Muscular Adaptations= | =Muscular Adaptations= | ||
− | * One of the most important adaptations to endurance training is the increase in the number of capillaries in the muscles. | + | * One of the most important adaptations to endurance training is the increase in the number of capillaries in the muscles. |
− | * Endurance training may increase the size of slow twitch (Type I) muscle fibers by up to 25%, or the training may decrease the size of slow twitch and some fast twitch (Type IIa) fibers<ref name="Trappe-2006">S. Trappe, M. Harber, A. Creer, P. Gallagher, D. Slivka, K. Minchev, D. Whitsett, Single muscle | + | * Endurance training may increase the size of slow twitch (Type I) muscle fibers by up to 25%, or the training may decrease the size of slow twitch and some fast twitch (Type IIa) fibers<ref name="Trappe-2006">S. Trappe, M. Harber, A. Creer, P. Gallagher, D. Slivka, K. Minchev, D. Whitsett, Single muscle [[Fiber]] adaptations with marathon training., J Appl Physiol, volume 101, issue 3, pages 721-7, Sep 2006, doi [http://dx.doi.org/10.1152/japplphysiol.01595.2005 10.1152/japplphysiol.01595.2005], PMID [http://www.ncbi.nlm.nih.gov/pubmed/16614353 16614353]</ref>. |
* There are changes within the fast twitch fibers so that Type IIb/IIx become more like Type IIa with endurance training. There is some evidence of a small change of fast twitch fibers to slow twitch. | * There are changes within the fast twitch fibers so that Type IIb/IIx become more like Type IIa with endurance training. There is some evidence of a small change of fast twitch fibers to slow twitch. | ||
− | * Oxygen is carried within a muscle | + | * Oxygen is carried within a muscle [[Fiber]] by myoglobin, which is similar to hemoglobin. Endurance training can increase the myoglobin in muscle fibers by up to 80%. |
− | * The size and number of mitochondria in the muscles increases with training. In rats, 27 weeks of exercise increased the mitochondria size by 35% and the number by 15%. | + | * The size and number of mitochondria in the muscles increases with training. In rats, 27 weeks of exercise increased the mitochondria size by 35% and the number by 15%. |
* Within the mitochondria, the oxidative enzymes increase with training. Over 7 months of swim training, one oxidative enzyme ([http://en.wikipedia.org/wiki/Succinate_dehydrogenase SDH]) increased by 800%. | * Within the mitochondria, the oxidative enzymes increase with training. Over 7 months of swim training, one oxidative enzyme ([http://en.wikipedia.org/wiki/Succinate_dehydrogenase SDH]) increased by 800%. | ||
=Metabolic Adaptations= | =Metabolic Adaptations= | ||
− | * Lactate | + | * [[Lactate Threshold]] is the exercise intensity at which [[Lactate]] starts to rapidly build up in the blood and is a key indicator of aerobic performance. Endurance training increases the exercise intensity of this threshold. |
− | * Endurance training results in a shift towards fat burning at sub-maximal exercise intensities. | + | * Endurance training results in a shift towards fat burning at sub-maximal exercise intensities. |
− | * [[Running Economy]] is improved by endurance training. At elite levels this may be a critical part of improving performance. | + | * [[Running Economy]] is improved by endurance training. At elite levels this may be a critical part of improving performance. |
− | * Maximum oxygen consumption ([[VO2max|V̇O<sub>2</sub>max]]) typically increases by 15-25% is typical for sedentary people who exercise for six months. The lower the initial [[VO2max|V̇O<sub>2</sub>max]], the greater the relative increase is likely to be. Typically an individual will reach their greatest [[VO2max|V̇O<sub>2</sub>max]] possible after 12-18 months. Longer term performance improvements come through being able to sustain higher percentages of [[VO2max|V̇O<sub>2</sub>max]] | + | * Maximum oxygen consumption ([[VO2max|V̇O<sub>2</sub>max]]) typically increases by 15-25% is typical for sedentary people who exercise for six months. The lower the initial [[VO2max|V̇O<sub>2</sub>max]], the greater the relative increase is likely to be. Typically an individual will reach their greatest [[VO2max|V̇O<sub>2</sub>max]] possible after 12-18 months. Longer term performance improvements come through being able to sustain higher percentages of [[VO2max|V̇O<sub>2</sub>max]] rather than increases in [[VO2max|V̇O<sub>2</sub>max]] . |
* Contrary to popular belief, endurance training does not raise the metabolic rate at rest. | * Contrary to popular belief, endurance training does not raise the metabolic rate at rest. | ||
+ | =Connective Tissue Adaptations= | ||
+ | In animal experiments, both endurance and resistance exercise results in improved tensile strength, elastic stiffness, weight and cross-sectional area of tendons<ref name="BaroneBellafiore2009"/>, and this has been confirmed in humans<ref name="LangbergRosendal2001"/>. While we tend to think of tendons as passive, mechanical structures, tendons consume glucose during exercise, just like muscles <ref name="HannukainenKalliokoski2005"/><ref name="Kalliokoski2005"/> (though to a lesser extent and the amount is not proportional to exercise intensity.) Research in runners has shown that a bout of running increases tendon growth. After 3 hours/36Km of running, the tendon growth markers decreased immediately post-run, but were 3-fold higher after 72 hours<ref name="LangbergSkovgaard1999"/>. Similar results were seen post-marathon, with a decrease immediately post-race, then rising to a peak after 3 days and returning to the baseline after 5 days<ref name="LangbergSkovgaard2014"/>. Shorter bursts of exercise have also shown increases in markers for tendon growth<ref name="BrahmPiehl-Aulin, S. Ljunghal1997"/><ref name="KristofferssonHultdin2007"/>. This suggests that running every day may hinder tendon healing. More evidence on the benefit of running comes from habitual runners that have larger tendons (cross sectional area) than non-runners, with studies measuring a 30-36% larger Achilles tendon<ref name="MagnussonKjaer2003"/><ref name="RosagerAagaard2002"/>. I didn't find any good studies that looked at the effect of training on tendons. One training program of running twice per week for 30-50 minutes at low intensity for 9 months did not increase Achilles tendon size<ref name="HansenAagaard2003"/>, but I suspect this is due to the relatively low volume of training. The benefits of exercise on tendon health is not limited to the young, with studies showing strengthening of tendons with exercise persists into old age<ref name="NariciMaganaris2005"/>. Perhaps not surprisingly, inactivity leads to a degradation of the tendons<ref name="MaganarisReeves2006"/>, hence the phrase "use it or lose it." | ||
=Time to Adapt= | =Time to Adapt= | ||
How long does it take to adapt to endurance training? | How long does it take to adapt to endurance training? | ||
Line 47: | Line 49: | ||
* Resting HR drops after 3 months with no further improvement thereafter<ref name="Scharhag-Rosenberger-2009"/> | * Resting HR drops after 3 months with no further improvement thereafter<ref name="Scharhag-Rosenberger-2009"/> | ||
* HR at a given submaximal intensity drops within 3 months<ref name="Scharhag-Rosenberger-2009"/> | * HR at a given submaximal intensity drops within 3 months<ref name="Scharhag-Rosenberger-2009"/> | ||
− | = Adaptations and Endurance Running= | + | =Adaptations and Endurance Running= |
[[File:Model of endurance running.jpg|none|thumb|500px|A model of how three adaptations impact endurance performance<ref name="Midgley-2007"/>.]] | [[File:Model of endurance running.jpg|none|thumb|500px|A model of how three adaptations impact endurance performance<ref name="Midgley-2007"/>.]] | ||
− | This flowchart shows that while [[VO2max|V̇O<sub>2</sub>max]] is a key determinant of performance, it interacts with other factors. Lactate threshold defines what percentage of [[VO2max|V̇O<sub>2</sub>max]] can be sustained, which defines the energy available (ATP re-synthesis). Running | + | This flowchart shows that while [[VO2max|V̇O<sub>2</sub>max]] is a key determinant of performance, it interacts with other factors. Lactate threshold defines what percentage of [[VO2max|V̇O<sub>2</sub>max]] can be sustained, which defines the energy available (ATP re-synthesis). [[Running Economy]] then defines how well this energy can be translated into forward motion. |
=References= | =References= | ||
<references> | <references> | ||
Line 56: | Line 58: | ||
<ref name="Scharhag-Rosenberger-2009">F. Scharhag-Rosenberger, T. Meyer, S. Walitzek, W. Kindermann, Time course of changes in endurance capacity: a 1-yr training study., Med Sci Sports Exerc, volume 41, issue 5, pages 1130-7, May 2009, doi [http://dx.doi.org/10.1249/MSS.0b013e3181935a11 10.1249/MSS.0b013e3181935a11], PMID [http://www.ncbi.nlm.nih.gov/pubmed/19346973 19346973]</ref> | <ref name="Scharhag-Rosenberger-2009">F. Scharhag-Rosenberger, T. Meyer, S. Walitzek, W. Kindermann, Time course of changes in endurance capacity: a 1-yr training study., Med Sci Sports Exerc, volume 41, issue 5, pages 1130-7, May 2009, doi [http://dx.doi.org/10.1249/MSS.0b013e3181935a11 10.1249/MSS.0b013e3181935a11], PMID [http://www.ncbi.nlm.nih.gov/pubmed/19346973 19346973]</ref> | ||
<ref name="Midgley-2007"> AW. Midgley, LR. McNaughton, AM. Jones, Training to enhance the physiological determinants of long-distance running performance: can valid recommendations be given to runners and coaches based on current scientific knowledge?, Sports Med, volume 37, issue 10, pages 857-80, 2007, PMID [http://www.ncbi.nlm.nih.gov/pubmed/17887811 17887811]</ref> | <ref name="Midgley-2007"> AW. Midgley, LR. McNaughton, AM. Jones, Training to enhance the physiological determinants of long-distance running performance: can valid recommendations be given to runners and coaches based on current scientific knowledge?, Sports Med, volume 37, issue 10, pages 857-80, 2007, PMID [http://www.ncbi.nlm.nih.gov/pubmed/17887811 17887811]</ref> | ||
+ | <ref name="BaroneBellafiore2009">R. Barone, M. Bellafiore, V. Leonardi, G. Zummo, Structural analysis of rat patellar tendon in response to resistance and endurance training, Scandinavian Journal of Medicine & Science in Sports, volume 19, issue 6, 2009, pages 782–789, ISSN [http://www.worldcat.org/issn/09057188 09057188], doi [http://dx.doi.org/10.1111/j.1600-0838.2008.00863.x 10.1111/j.1600-0838.2008.00863.x]</ref> | ||
+ | <ref name="LangbergSkovgaard1999">Henning Langberg, Dorthe Skovgaard, Lars J. Petersen, Jens Bülow, Michael Kjaer, Type I collagen synthesis and degradation in peritendinous tissue after exercise determined by microdialysis in humans, The Journal of Physiology, volume 521, issue 1, 1999, pages 299–306, ISSN [http://www.worldcat.org/issn/00223751 00223751], doi [http://dx.doi.org/10.1111/j.1469-7793.1999.00299.x 10.1111/j.1469-7793.1999.00299.x]</ref> | ||
+ | <ref name="LangbergRosendal2001">Henning Langberg, Lars Rosendal, Michael Kjaer, Training-induced changes in peritendinous type I collagen turnover determined by microdialysis in humans, The Journal of Physiology, volume 534, issue 1, 2001, pages 297–302, ISSN [http://www.worldcat.org/issn/00223751 00223751], doi [http://dx.doi.org/10.1111/j.1469-7793.2001.00297.x 10.1111/j.1469-7793.2001.00297.x]</ref> | ||
+ | <ref name="HannukainenKalliokoski2005">J. Hannukainen, K. K. Kalliokoski, P. Nuutila, T. Fujimoto, J. Kemppainen, T. Viljanen, M. S. Laaksonen, R. Parkkola, J. Knuuti, M. Kjær, In Vivo Measurements of Glucose Uptake in Human Achilles Tendon During Different Exercise Intensities, International Journal of Sports Medicine, volume 26, issue 9, 2005, pages 727–731, ISSN [http://www.worldcat.org/issn/0172-4622 0172-4622], doi [http://dx.doi.org/10.1055/s-2005-837458 10.1055/s-2005-837458]</ref> | ||
+ | <ref name="Kalliokoski2005">K. K. Kalliokoski, The effect of dynamic knee-extension exercise on patellar tendon and quadriceps femoris muscle glucose uptake in humans studied by positron emission tomography, Journal of Applied Physiology, volume 99, issue 3, 2005, pages 1189–1192, ISSN [http://www.worldcat.org/issn/8750-7587 8750-7587], doi [http://dx.doi.org/10.1152/japplphysiol.00283.2005 10.1152/japplphysiol.00283.2005]</ref> | ||
+ | <ref name="MaganarisReeves2006">Constantinos N. Maganaris, Neil D. Reeves, Joern Rittweger, Anthony J. Sargeant, David A. Jones, Karin Gerrits, Arnold De Haan, Adaptive response of human tendon to paralysis, Muscle & Nerve, volume 33, issue 1, 2006, pages 85–92, ISSN [http://www.worldcat.org/issn/0148-639X 0148-639X], doi [http://dx.doi.org/10.1002/mus.20441 10.1002/mus.20441]</ref> | ||
+ | <ref name="NariciMaganaris2005">M.V. Narici, C. Maganaris, N. Reeves, Myotendinous alterations and effects of resistive loading in old age, Scandinavian Journal of Medicine and Science in Sports, volume 15, issue 6, 2005, pages 392–401, ISSN [http://www.worldcat.org/issn/0905-7188 0905-7188], doi [http://dx.doi.org/10.1111/j.1600-0838.2005.00458.x 10.1111/j.1600-0838.2005.00458.x]</ref> | ||
+ | <ref name="RosagerAagaard2002">S. Rosager, P. Aagaard, P. Dyhre-Poulsen, K. Neergaard, M. Kjaer, S. P. Magnusson, Load-displacement properties of the human triceps surae aponeurosis and tendon in runners and non-runners, Scandinavian Journal of Medicine and Science in Sports, volume 12, issue 2, 2002, pages 90–98, ISSN [http://www.worldcat.org/issn/0905-7188 0905-7188], doi [http://dx.doi.org/10.1034/j.1600-0838.2002.120205.x 10.1034/j.1600-0838.2002.120205.x]</ref> | ||
+ | <ref name="MagnussonKjaer2003">S. Peter Magnusson, Michael Kjaer, Region-specific differences in Achilles tendon cross-sectional area in runners and non-runners, European Journal of Applied Physiology, volume 90, issue 5-6, 2003, pages 549–553, ISSN [http://www.worldcat.org/issn/1439-6319 1439-6319], doi [http://dx.doi.org/10.1007/s00421-003-0865-8 10.1007/s00421-003-0865-8]</ref> | ||
+ | <ref name="HansenAagaard2003">P. Hansen, P. Aagaard, M. Kjaer, B. Larsson, S. P. Magnusson, Effect of habitual running on human Achilles tendon load-deformation properties and cross-sectional area, Journal of Applied Physiology, volume 95, issue 6, 2003, pages 2375–2380, ISSN [http://www.worldcat.org/issn/8750-7587 8750-7587], doi [http://dx.doi.org/10.1152/japplphysiol.00503.2003 10.1152/japplphysiol.00503.2003]</ref> | ||
+ | <ref name="LangbergSkovgaard2014">H. Langberg, D. Skovgaard, S. Asp, M. Kjær, Time Pattern of Exercise-Induced Changes in Type I Collagen Turnover after Prolonged Endurance Exercise in Humans, Calcified Tissue International, volume 67, issue 1, 2014, pages 41–44, ISSN [http://www.worldcat.org/issn/0171-967X 0171-967X], doi [http://dx.doi.org/10.1007/s00223001094 10.1007/s00223001094]</ref> | ||
+ | <ref name="KristofferssonHultdin2007">A. Kristoffersson, J. Hultdin, I. Holmlund, K. Thorsen, R. Lorentzon, Effects of Short-Term Maximal Work on Plasma Calcium, Parathyroid Hormone, Osteocalcin and Biochemical Markers of Collagen Metabolism, International Journal of Sports Medicine, volume 16, issue 03, 2007, pages 145–149, ISSN [http://www.worldcat.org/issn/0172-4622 0172-4622], doi [http://dx.doi.org/10.1055/s-2007-972982 10.1055/s-2007-972982]</ref> | ||
+ | <ref name="BrahmPiehl-Aulin, S. Ljunghal1997">H. Brahm, K. Piehl-Aulin, S. Ljunghal, Bone Metabolism During Exercise and Recovery: The Influence of Plasma Volume and Physical Fitness, Calcified Tissue International, volume 61, issue 3, 1997, pages 192–198, ISSN [http://www.worldcat.org/issn/0171-967X 0171-967X], doi [http://dx.doi.org/10.1007/s002239900322 10.1007/s002239900322]</ref> | ||
</references> | </references> | ||
+ | [[Category:Advanced]] | ||
+ | [[Category:Science]] |
Latest revision as of 15:15, 22 December 2016
There are various adaptations to endurance training, which are discussed in some detail below. Understanding these adaptations can be useful background when looking at the different types of training. (Except where noted, this list is based on "Physiology of sport and exercise"[1].)
Contents
1 Heart Adaptations
- The heart becomes larger in response to endurance training, with the left side that sends blood to the body having a thicker wall and a larger interior volume.
- The stroke volume (blood pumped with each beat) also increases. The volume is greater at rest and during exercise, with the stroke volume in a highly trained athlete twice that of an untrained person. This increase is due to multiple factors:
- The increased heart size.
- A slower Heart Rate for a given exercise level which allows more time for the heart to fill.
- Increased total blood volume.
- A greater force of contraction.
- A lower volume of blood remaining in the heart after contraction.
- Resting Heart Rate drops with training. A sedentary person with a resting Heart Rate of 80 BPM may be able to reduce their resting Heart Rate by 1 BPM per week of training for up to 10 weeks, though some studies have shown much smaller changes (5 BPM over 20 weeks).
- The Heart Rate for a given exercise intensity is reduced, and sedentary people undergoing 6 months of training can reduce the Heart Rate for a given intensity by 10-30 BPM.
- Maximum Heart Rate does not typically change with endurance training, but several studies have suggested that sedentary people with a Max HR of 180+ BPM may have a slightly reduced value post-training. This reduction in Max HR may be to preserve the time for the heart to refill with blood. Also, older athletes may have less of an age related decline in Max HR than those that are sedentary.
- Cardiac output at rest and at moderate intensity exercise remains largely unchanged with training, which is the result of a greater stroke volume at a lower Heart Rate. (Sometimes the cardiac output will be slightly lower, probably due to greater oxygen extraction by the muscles.) However, at higher intensity exercise the cardiac output is increased, which results in improved performance.
2 Circulation Adaptations
- Endurance training results in an increased number of capillaries in the muscles. The ratio of capillaries to muscle fibers allows for a greater delivery of blood to the working muscles. In addition, the use of existing capillaries is improved with training.
- The human body does not have enough blood to fully supply all capillaries in the body, and so it will redistribute the blood flow to the most needed areas. Endurance training improves this redistribution, including redistribution within the muscles themselves, providing more blood to the slow twitch fibers and less to the fast twitch fibers.
- Blood pressure at a given exercise intensity is reduced, but at maximum intensity systolic pressure is increased and diastolic is reduced. (Systolic is the higher pressure when the heart beats and diastolic is the lower pressure when the heart is refilling.)
- Endurance training generally lowers resting blood pressure. Systolic is reduced by ~4 mm Hg and diastolic by 3 mm Hg in people with either high or normal blood pressure[2].
- Blood volume increases with endurance training, and higher intensity produces a greater change.
- Blood plasma increases rapidly, with initial changes seen within an hour of the end of the first bout of training.
- The number of red blood cells, that carry oxygen in the blood, also increases with training. While these extra cells add to the increase in overall blood volume, because the plasma increases disproportionately more than the red blood cells, the net effect is that the blood becomes more dilute and can result in a false indication of anemia (pseudoanemia). Having thinner blood may be an advantage to an athlete, as it will flow more easily through the capillaries.
- The oxygen saturation of blood returning from the exercising body to the heart is lowered after training. This is due to an improved ability of the muscles to utilize the supplied oxygen
3 Lung (pulmonary) Adaptations
- Generally, there is little structural adaptation of the lungs, as their capacity is far greater than is needed in most individuals.
- Breathing at rest is unchanged with training, but for a given sub-maximal intensity, Breathing is reduced by as much as 20-30%. Maximal Breathing increases with training, with increases in both the Breathing rate and volume.
- In some highly trained athletes the lungs are unable to keep up at high exercise intensities and the blood oxygen saturation (SpO2) can drop.
- Training increases the blood flow to the lungs (perfusion), which combined with greater Breathing and lower oxygen saturation of the blood entering the lungs results in a greater uptake of oxygen (pulmonary diffusion).
4 Muscular Adaptations
- One of the most important adaptations to endurance training is the increase in the number of capillaries in the muscles.
- Endurance training may increase the size of slow twitch (Type I) muscle fibers by up to 25%, or the training may decrease the size of slow twitch and some fast twitch (Type IIa) fibers[3].
- There are changes within the fast twitch fibers so that Type IIb/IIx become more like Type IIa with endurance training. There is some evidence of a small change of fast twitch fibers to slow twitch.
- Oxygen is carried within a muscle Fiber by myoglobin, which is similar to hemoglobin. Endurance training can increase the myoglobin in muscle fibers by up to 80%.
- The size and number of mitochondria in the muscles increases with training. In rats, 27 weeks of exercise increased the mitochondria size by 35% and the number by 15%.
- Within the mitochondria, the oxidative enzymes increase with training. Over 7 months of swim training, one oxidative enzyme (SDH) increased by 800%.
5 Metabolic Adaptations
- Lactate Threshold is the exercise intensity at which Lactate starts to rapidly build up in the blood and is a key indicator of aerobic performance. Endurance training increases the exercise intensity of this threshold.
- Endurance training results in a shift towards fat burning at sub-maximal exercise intensities.
- Running Economy is improved by endurance training. At elite levels this may be a critical part of improving performance.
- Maximum oxygen consumption (V̇O2max) typically increases by 15-25% is typical for sedentary people who exercise for six months. The lower the initial V̇O2max, the greater the relative increase is likely to be. Typically an individual will reach their greatest V̇O2max possible after 12-18 months. Longer term performance improvements come through being able to sustain higher percentages of V̇O2max rather than increases in V̇O2max .
- Contrary to popular belief, endurance training does not raise the metabolic rate at rest.
6 Connective Tissue Adaptations
In animal experiments, both endurance and resistance exercise results in improved tensile strength, elastic stiffness, weight and cross-sectional area of tendons[4], and this has been confirmed in humans[5]. While we tend to think of tendons as passive, mechanical structures, tendons consume glucose during exercise, just like muscles [6][7] (though to a lesser extent and the amount is not proportional to exercise intensity.) Research in runners has shown that a bout of running increases tendon growth. After 3 hours/36Km of running, the tendon growth markers decreased immediately post-run, but were 3-fold higher after 72 hours[8]. Similar results were seen post-marathon, with a decrease immediately post-race, then rising to a peak after 3 days and returning to the baseline after 5 days[9]. Shorter bursts of exercise have also shown increases in markers for tendon growth[10][11]. This suggests that running every day may hinder tendon healing. More evidence on the benefit of running comes from habitual runners that have larger tendons (cross sectional area) than non-runners, with studies measuring a 30-36% larger Achilles tendon[12][13]. I didn't find any good studies that looked at the effect of training on tendons. One training program of running twice per week for 30-50 minutes at low intensity for 9 months did not increase Achilles tendon size[14], but I suspect this is due to the relatively low volume of training. The benefits of exercise on tendon health is not limited to the young, with studies showing strengthening of tendons with exercise persists into old age[15]. Perhaps not surprisingly, inactivity leads to a degradation of the tendons[16], hence the phrase "use it or lose it."
7 Time to Adapt
How long does it take to adapt to endurance training?
- V̇O2max, cardiac output, stroke volume changes start within 3 weeks, but continue linearly for at least 12 weeks in both old and young[17].
- V̇O2max continues to improve for at least 12 months[18]
- Resting HR drops after 3 months with no further improvement thereafter[18]
- HR at a given submaximal intensity drops within 3 months[18]
8 Adaptations and Endurance Running
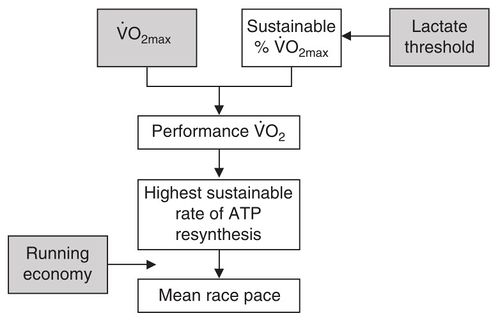
This flowchart shows that while V̇O2max is a key determinant of performance, it interacts with other factors. Lactate threshold defines what percentage of V̇O2max can be sustained, which defines the energy available (ATP re-synthesis). Running Economy then defines how well this energy can be translated into forward motion.
9 References
- ↑ Jack H. Wilmore, David L. Costill, W. Larry. Kenney, Physiology of sport and exercis, 2008 !!date!!, Human Kinetics !!publisher!!, Champaign, IL !!location!!, isbn 0-7360-5583-5
- ↑ SP. Whelton, A. Chin, X. Xin, J. He, Effect of aerobic exercise on blood pressure: a meta-analysis of randomized, controlled trials., Ann Intern Med, volume 136, issue 7, pages 493-503, Apr 2002, PMID 11926784
- ↑ S. Trappe, M. Harber, A. Creer, P. Gallagher, D. Slivka, K. Minchev, D. Whitsett, Single muscle Fiber adaptations with marathon training., J Appl Physiol, volume 101, issue 3, pages 721-7, Sep 2006, doi 10.1152/japplphysiol.01595.2005, PMID 16614353
- ↑ R. Barone, M. Bellafiore, V. Leonardi, G. Zummo, Structural analysis of rat patellar tendon in response to resistance and endurance training, Scandinavian Journal of Medicine & Science in Sports, volume 19, issue 6, 2009, pages 782–789, ISSN 09057188, doi 10.1111/j.1600-0838.2008.00863.x
- ↑ Henning Langberg, Lars Rosendal, Michael Kjaer, Training-induced changes in peritendinous type I collagen turnover determined by microdialysis in humans, The Journal of Physiology, volume 534, issue 1, 2001, pages 297–302, ISSN 00223751, doi 10.1111/j.1469-7793.2001.00297.x
- ↑ J. Hannukainen, K. K. Kalliokoski, P. Nuutila, T. Fujimoto, J. Kemppainen, T. Viljanen, M. S. Laaksonen, R. Parkkola, J. Knuuti, M. Kjær, In Vivo Measurements of Glucose Uptake in Human Achilles Tendon During Different Exercise Intensities, International Journal of Sports Medicine, volume 26, issue 9, 2005, pages 727–731, ISSN 0172-4622, doi 10.1055/s-2005-837458
- ↑ K. K. Kalliokoski, The effect of dynamic knee-extension exercise on patellar tendon and quadriceps femoris muscle glucose uptake in humans studied by positron emission tomography, Journal of Applied Physiology, volume 99, issue 3, 2005, pages 1189–1192, ISSN 8750-7587, doi 10.1152/japplphysiol.00283.2005
- ↑ Henning Langberg, Dorthe Skovgaard, Lars J. Petersen, Jens Bülow, Michael Kjaer, Type I collagen synthesis and degradation in peritendinous tissue after exercise determined by microdialysis in humans, The Journal of Physiology, volume 521, issue 1, 1999, pages 299–306, ISSN 00223751, doi 10.1111/j.1469-7793.1999.00299.x
- ↑ H. Langberg, D. Skovgaard, S. Asp, M. Kjær, Time Pattern of Exercise-Induced Changes in Type I Collagen Turnover after Prolonged Endurance Exercise in Humans, Calcified Tissue International, volume 67, issue 1, 2014, pages 41–44, ISSN 0171-967X, doi 10.1007/s00223001094
- ↑ H. Brahm, K. Piehl-Aulin, S. Ljunghal, Bone Metabolism During Exercise and Recovery: The Influence of Plasma Volume and Physical Fitness, Calcified Tissue International, volume 61, issue 3, 1997, pages 192–198, ISSN 0171-967X, doi 10.1007/s002239900322
- ↑ A. Kristoffersson, J. Hultdin, I. Holmlund, K. Thorsen, R. Lorentzon, Effects of Short-Term Maximal Work on Plasma Calcium, Parathyroid Hormone, Osteocalcin and Biochemical Markers of Collagen Metabolism, International Journal of Sports Medicine, volume 16, issue 03, 2007, pages 145–149, ISSN 0172-4622, doi 10.1055/s-2007-972982
- ↑ S. Peter Magnusson, Michael Kjaer, Region-specific differences in Achilles tendon cross-sectional area in runners and non-runners, European Journal of Applied Physiology, volume 90, issue 5-6, 2003, pages 549–553, ISSN 1439-6319, doi 10.1007/s00421-003-0865-8
- ↑ S. Rosager, P. Aagaard, P. Dyhre-Poulsen, K. Neergaard, M. Kjaer, S. P. Magnusson, Load-displacement properties of the human triceps surae aponeurosis and tendon in runners and non-runners, Scandinavian Journal of Medicine and Science in Sports, volume 12, issue 2, 2002, pages 90–98, ISSN 0905-7188, doi 10.1034/j.1600-0838.2002.120205.x
- ↑ P. Hansen, P. Aagaard, M. Kjaer, B. Larsson, S. P. Magnusson, Effect of habitual running on human Achilles tendon load-deformation properties and cross-sectional area, Journal of Applied Physiology, volume 95, issue 6, 2003, pages 2375–2380, ISSN 8750-7587, doi 10.1152/japplphysiol.00503.2003
- ↑ M.V. Narici, C. Maganaris, N. Reeves, Myotendinous alterations and effects of resistive loading in old age, Scandinavian Journal of Medicine and Science in Sports, volume 15, issue 6, 2005, pages 392–401, ISSN 0905-7188, doi 10.1111/j.1600-0838.2005.00458.x
- ↑ Constantinos N. Maganaris, Neil D. Reeves, Joern Rittweger, Anthony J. Sargeant, David A. Jones, Karin Gerrits, Arnold De Haan, Adaptive response of human tendon to paralysis, Muscle & Nerve, volume 33, issue 1, 2006, pages 85–92, ISSN 0148-639X, doi 10.1002/mus.20441
- ↑ JM. Murias, JM. Kowalchuk, DH. Paterson, Time course and mechanisms of adaptations in cardiorespiratory fitness with endurance training in older and young men., J Appl Physiol, volume 108, issue 3, pages 621-7, Mar 2010, doi 10.1152/japplphysiol.01152.2009, PMID 20056848
- ↑ 18.0 18.1 18.2 F. Scharhag-Rosenberger, T. Meyer, S. Walitzek, W. Kindermann, Time course of changes in endurance capacity: a 1-yr training study., Med Sci Sports Exerc, volume 41, issue 5, pages 1130-7, May 2009, doi 10.1249/MSS.0b013e3181935a11, PMID 19346973
- ↑ RC. Hickson, JM. Hagberg, AA. Ehsani, JO. Holloszy, Time course of the adaptive responses of aerobic power and heart rate to training., Med Sci Sports Exerc, volume 13, issue 1, pages 17-20, 1981, PMID 7219130
- ↑ AW. Midgley, LR. McNaughton, AM. Jones, Training to enhance the physiological determinants of long-distance running performance: can valid recommendations be given to runners and coaches based on current scientific knowledge?, Sports Med, volume 37, issue 10, pages 857-80, 2007, PMID 17887811
- Category:Advanced
- Category:Science